When it comes to designing improved materials for components and structures of aerospace engines, most of the attention is focused on how to reduce the fuel consumption of the engine for both environmental and economical reasons.
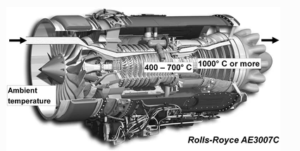
There are two main approaches to decrease the fuel consumption of an aerospace engine:
1st: Decreasing the mass of the structure.
The lighter the structure is, the less energy is required to move it. In order to decrease the mass of the structure, we aim for materials with low density such as Titanium and Nickel alloys (4 – 8 g/cm3) which are widely used in aerospace for their very good combination of mechanical properties and low densities. We can also aim to improve the mechanical properties of the materials used (Yield strength and ductility, fatigue resistance…), which then allows us to build more mater-efficient engine parts.
2nd: Improving the engine efficiency.
The efficiency of an aerospace engine can be assimilated to a thermal engine efficiency, since the amounts of heat & work generated are directly linked to the amount of fuel consumed. The maximum Carnot efficiency achievable is given by the following relationship:
Where Tc and Th are respectively the temperatures of the cold and hot sources. This equation shows that in order to maximise the engine efficiency ηcmax, we need to increase Th, the working temperature of the engine.
The critical part of the engine, undergoing the maximum amount of heat and stress are the gas turbines (figure 1). It is this part of the engine that currently limits the operating temperature, as the materials used are pushed to their limit in terms of creep strength, melting point and high temperature mechanical performance.
– Alloys studied –
Nickel superalloys are currently the best performing materials for gas turbine applications. Their exceptional high temperature performance is attributed to their dual phase microstructure, consisting in a disordered gamma matrix with ordered gamma prime precipitates. Here we explore the potential of titanium-based alloys composed of a bcc (A2) matrix with ordered bcc (B2) precipitates. The idea is that mimicking the Nickel superalloy microstructure should lead to good high temperature performance, and doing it using a bcc titanium matrix allows to exploit the low density of titanium combined with refractory bcc stabilising elements such as Mo and Nb. This could lead to overall better performing alloys, both in terms of density and high temperature performance.
One early example of such alloys lies in the Ti-Fe-Mo alloy system, exploiting a bcc Ti matrix with B2 TiFe intermetallic precipitates:
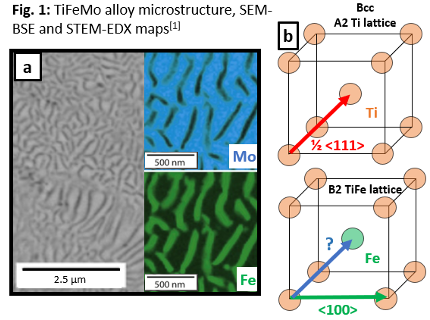
Fig 1: a) A Ti-Fe-Mo bcc+B2 alloy microstructure and EDX mapping ([1] http://dx.doi.org/10.1016/j.scriptamat.2017.06.038) b) Associated crystal geometry and favoured slip systems of bcc and B2 phases.
These alloys showed the targeted microstructural features as well as interesting recrystallisation mechanism which are still under study.
One key challenge for the development of these “bcc-superalloys” is the question of ductility. The bcc lattice is often described as an inherently brittle crystal structure. Combining it with B2 precipitates complexifies the situation further as it adds concerns about load partitioning and strain accumulation between the phases:
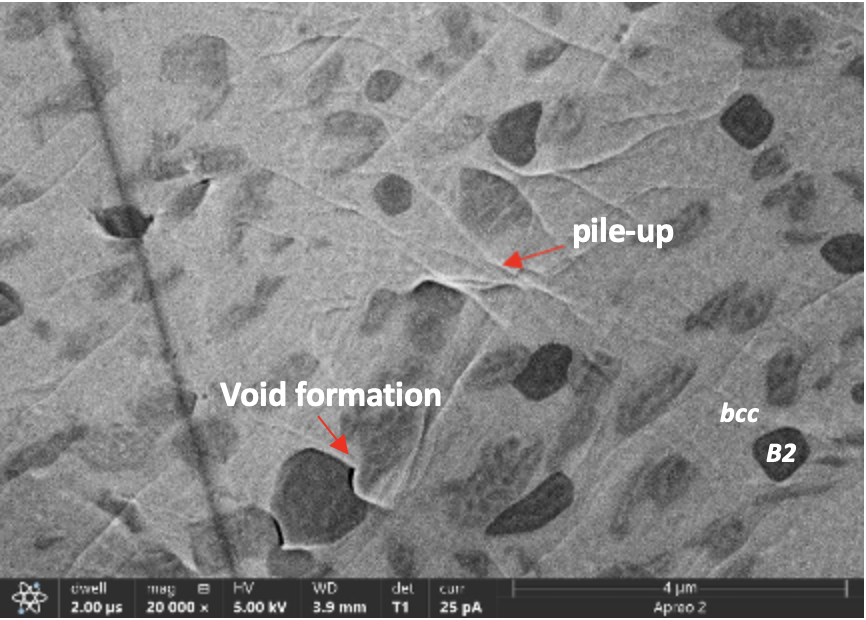
Fig 2: microhardness indentation induced dislocation motion in a Ti-Fe-Nb bcc+B2 alloy.
Therefore, multiple strategies are being studied to achieve better ductility:
- the use of Nb which is known to ductilise titanium alloys,
- the softening of the B2 precipitates to promote shearing mechanisms and avoid strain accumulations
- the control of the slip activity in the B2 for better compatibility in slip systems between the matrix and the precipitates.
Some alloying elements allowed clear improvements in ductility over the Ti-Fe-Mo system. Al addition help reducing the misfit between the phases and soften the B2 TiFe phase promoting precipitate shearing. Ti-Fe-Nb-Al systems showed significantly more ductile deformation modes at the micro-scale, with good phase control and precipitate shearing. Work is on its way to test them at larger scale and higher temperatures.